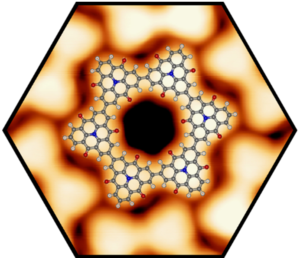
On-surface covalent chemistry represents a potent alternative for the bottom-up construction of various carbon-based architectures, which are otherwise inaccessible through conventional synthetic methods. This process can also be monitored by scanning probe microscopy techniques. Moreover, the approach appears to be particularly attractive in the context of carbon-based molecular electronics, since the respective nanostructures can be fabricated directly on a surface. This field is currently experiencing a dynamic expansion, as our team and others alike recently demonstrated the rational construction of nanographenes, nanoribbons, fullerenes, and nanotubes. In the first and second funding periods we have accomplished various families of successful concepts for on-surface SCA chemistry. We have, for example, considerably broadened the access to new precursor molecules for on-surface transformation to SCAs and other carbon-rich nanostructures. For example, we have already achieved highly-ordered, two-dimensional, covalent networks pores, and provided the initial evidence for the on-surface synthesis of graphyrins. We have also provided fundamental contributions towards topological covalent chemistry of single-layer graphene deposited on various surfaces. Based on these pioneering results on-surface supported carbon architectures (isocylic and heterocyclic), we will carry the highly attractive opportunities for surface-mediated SCA chemistry (0D, 1D, and 2D) a considerable step forward. We are now in the position to manufacture various families of hemispherical buckybowls (nanotube seeds), graphenoid nanoribbons, carbon nanotubes of defined chirality, porous 2D-heterographenes, and other atomically defined carbon nano-architectures. At the same time, we will accomplish the spatially resolved covalent surface patterning of graphene which will open a new chapter in the field of SCA research.